Research
The goal of the Tyler lab is to discover and understand the mechanistic interplay between epigenetics, genomic integrity and aging. We perform hypothesis driven research, but are most excited to follow unexpected results not predicted by our hypotheses, taking us in unanticipated new research directions. Our studies use a combination of molecular genetics in budding yeast, mammalian tissue culture, CRISPR/Cas9 gRNA genetic screens, and biochemical approaches. The proteins and processes that we study are so highly conserved through eukaryotic evolution, that what we learn using highly genetically malleable systems, including budding yeast, is directly relevant to the situation in humans. For example, the histone proteins that package our genome into chromatin are the most highly conserved protein in all eukaryotic evolution, indicating the profound importance of their functions.
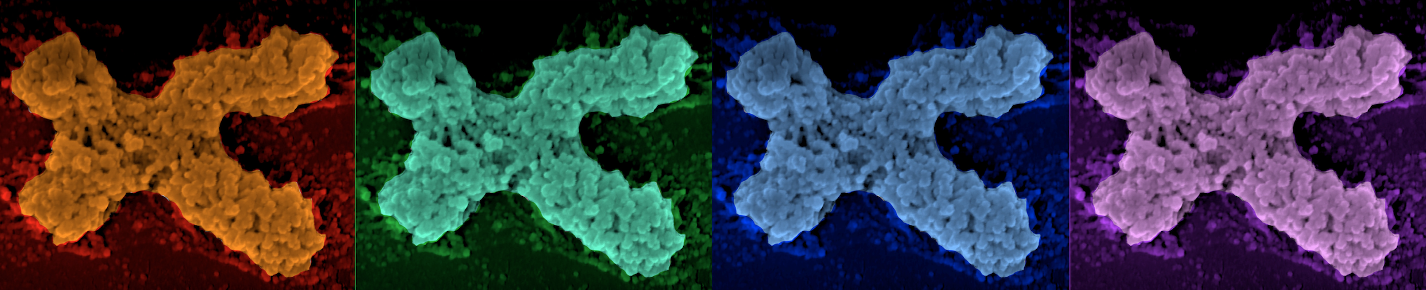 |
Our lab made significant contributions to the knowledge that depositing histones onto the genome, removing histones from the genome, and histone post-translational modifications are utilized by the cell to enable an exquisite level of regulation of gene expression, genome stability (specifically DNA double-strand repair), DNA replication and chromosome structure (above). In addition to learning how chromatin regulates fundamental processes in the cell, our studies are revealling how defects in the chromatin structure lead to gene dysfunction and genomic instability, which in turn are the cause of human aging and disease states including cancer and leukemia. For example, we discovered that histone packaging of the genome is strikingly reduced during aging, while experimental overexpression of histones extends longevity.
Our fascination with aging and longevity stimulated our comprehensive characterization of the aging process in budding yeast. Budding yeast is the only cell type where the number of cell divisions in a lifespan, mitotic / replicative lifespan, can be accurately measured and is a model for stem cell aging. It was well established that mutations or human diseases that cause genome instability shorten lifespan. However, it was not clear if improved DNA repair can extend lifespan. We found that at least one reason why the genome becomes progressively unstable during aging is due to defective DNA repair by homologous recombination. Moreover, overexpressing proteins required for homologous recombination significantly improved DNA repair in old cells, leading to extended lifespan. We predict that interventions to improve DNA double-strand break repair will also extend lifespan in mammals.
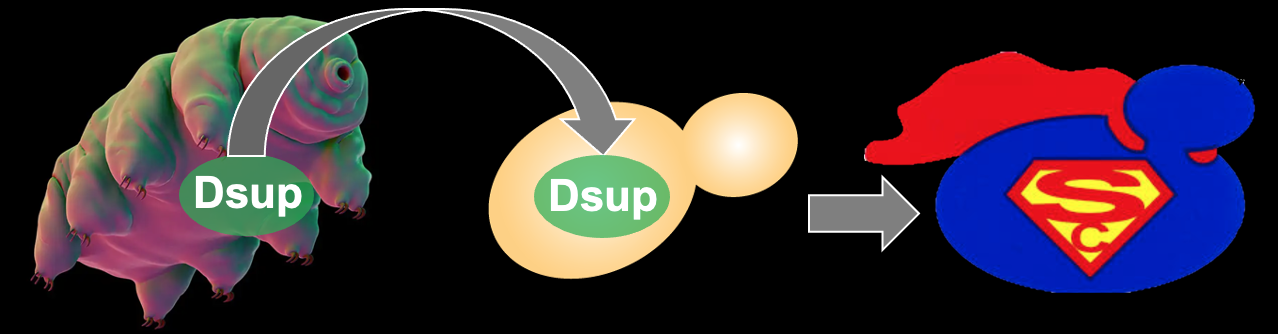 |
Our fascination with the interplay between epigenetics, genomic integrity and aging recently led us to express a Tardigrade protein, Dsup, in budding yeast, in an attempt to make "super yeast". Dsup is considered to be at least partly responsible for the ability of some Tardigrade species to withstand doses of irradiation 1000 times higher than doses lethal to humans. Upon exposure to oxidative DNA damage, the "super yeast" lived longer and their genomes were protected from DNA damage; this occurred in a manner dependent on Dsup coating the entire genome via multivalent interactions with histones and DNA.
These and other studies utilize cutting edge technologies including microfluidics platforms to fluorescently image individual cells throughout their lifespan to interrogate how critical cellular properties, such as genome stability, decay at the molecular level during aging. We are identifying genetic and environmental regiments to reduce the decline of key cellular processes during aging, with the ultimate goal to leverage our discoveries to therapeutics to extend human lifespan and health span.